(3) Determine the net work output and the
thermal efficiency of the ideal Brayton cycle with intercooling, reheating and regeneration
The T-s diagram of the ideal Brayton cycle with intercooling, reheating, and regeneration is shown on the left.
Under the cold-air-standard assumption, heat input is the sum of the
enthalpy differences in the constant-pressure heat addition process and the reheating process. Heat output equals
the sum of the enthalpy differences in the constant-pressure heat rejection process and the intercooling process.
qin = (h6 - h5) + (h8 -
h7)
= cP(T6-
T5) + cP(T8- T7)
qout = (h10 -
h1) + (h2 - h3)
= cP(T10- T1) + cP(T2-
T3)
The temperature at each state can be determined by the same way as in (1) and (2).
T1 = 300 K (given)
P1 = 100 kPa (given)
For optimum operation, equal pressure ratios are maintained across each
state.

The total pressure ratio is given as rP = 8
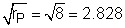
P2 = 2.828P1= 282.8 kPa
1-2: isentropic compression:
T2 = T1(P2/P1)(k-1)/k =
403.8 K
2-3: constant pressure intercooling. For optimum operation, air is cooled
to a temperature same as the temperature at the compressor inlet.
Hence,
T3 = T1 = 300 K
P3 = P2 = 282.8
kPa
3-4: isentropic compression:
P4 = 2.828P3 =
800 kPa
T4 = T3(P4/P3)(k-1)/k =
403.8 K
Temperature at state 6 reaches the maximum value. The temperature is
given as
T6 = 1,300 K
4-5-6: constant pressure process:
P6 = P4 = 800 kPa
6-7: isentropic expansion:
P7 = P6 /2.828 = 282.8 kPa
T7 = T6(P7/P6)(k-1)/k =
965.9 K
7-8: constant pressure reheating process:
P8 = P7 = 282.8 kPa
For optimum operation, air is heated to the same temperature at each
inlet of the turbine. Hence,
T8 = T6 =
1,300 K
9-10-1: constant pressure process:
P9 = P1 = 100 kPa
8-9: isentropic expansion:
T9 = T8(P9/P8)(k-1)/k = 965.9 K
4-5 and 9-10: constant pressure regeneration:
|